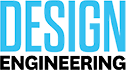
Researchers develop NDT technique to analyze defects in 2D materials
Staff
Quality NDTThis method can be very helpful in establishing a protocol for characterization of 2D crystalline materials.
2D materials are becoming more commonplace in electronics, sensing, early cancer diagnosis tools, water desalination and a range of other unique applications. Thinning a material down to a single atom thickness can drastically modify its physical properties.
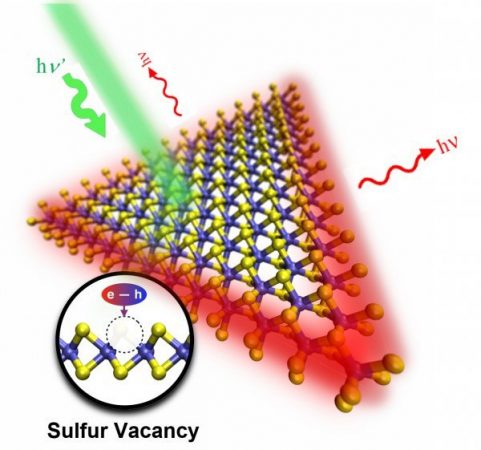
Molecular model of a WS2 triangular monolayer targeted with a green laser (hv’). Red light (hv) is emitted from the edges where defects consisting of sulfur vacancies are located. Electron-hole pairs are bound at the vacancy site (see inset). Credit: Yuanxi Wang, Penn State
A team of Penn State researchers in the Department of Physics and the Center for Two-Dimensional and Layered Materials (2DLM) has developed a fast, non-destructive optical method for analyzing defects in these two-dimensional materials.
“In the semiconductor industry, for example, defects are important because you can control properties through defects,” said Mauricio Terrones, professor of physics, materials science and engineering and chemistry, Penn State. “This is known as defect engineering. Industry knows how to control defects and which types are good for devices.”
To really understand what is going on in a 2D material like tungsten disulfide, which has a single atom-thick layer of tungsten sandwiched between two atomic layers of sulfur, would require a high-power electron microscope capable of seeing individual atoms and the holes, called vacancies, where the atoms are missing.
“The benefit of transmission electron microscopy (TEM) is that you get an image and you can see directly what is going on – you get direct evidence,” said Bernd Kabius, staff scientist at Penn State’s Materials Research Institute, an expert in TEM.
There are some downsides, however. According to Kabius, this method increases the possibility of damage to the delicate 2D material, requires complex preparation of the sample and a can be extremely time consuming. This method can measure up to an entire day of instrument time to image a single sample and a week or more to interpret the results.
For those reasons, and others, researchers would like to combine TEM with another method of looking at the sample that is simpler and faster.
The technique developed by Terrones and his team uses an optical method, fluorescent microscopy, in which a laser of a specific wavelength is shone on a sample and the excited electrons, pushed to a higher energy level, each emit a photon of a longer wavelength when the electron drops down to a lower energy level.
The wavelength, or color of light, can be measured by spectroscopy and gives information about the defect type and location on the sample. This data shows up as peaks on a graph, which the team then correlated to visual confirmation under the TEM. Theoretical calculations also helped to validate the optical results.
A necessary step in the process requires placing the sample in a temperature-controlled specimen holder, or stage, and lowering the temperature to 77 kelvin, almost 200 degrees C below zero. At this temperature, the electron-hole pairs that produce the fluorescence are bound to the defect and emit a signal stronger than the pristine areas of the material.
“For the first time, we have established a direct relationship between the optical response and the amount of atomic defects in two-dimensional materials,” said Victor Carozo, former postdoctoral scholar in Terrones’ lab and first author of the work.
“We can establish not just an empirical correlation between the presence of certain defects and modified light emission, but also identify the reason for that correlation through first-principles calculations,” explains Vincent Crespi, Distinguished Professor of Physics, Materials Science and Engineering and Chemistry, Penn State.
Torrones believes that this method can be very helpful in establishing a protocol for characterization of 2D crystalline materials.
In addition to Terrones, Carozo, Kabius and Crespi, authors on the Science Advances paper, titled “Optical Identification of Sulfur Vacancies: Bound Excitons at the Edges of Monolayer Tungsten Disulfide,” are postdoctoral scholars Yuanxi Wang, Kazunori Fujisawa, Bruno Carvalho and Amber McCreary; Ph.D. students Simin Feng, Zhong Lin and Chanjing Zhou; research associates Nestor Perea-Lopez and Ana Laura Elias. All of the work was performed at Penn State.